Location
Physics : 401
Date & Time
July 31, 2017, 10:00 am – 11:00 am
Description
ADVISOR: Dr. Katja Pottschmidt
TITLE: Shedding New Light on Accreting Pulsars.
ABSTRACT: Neutron stars are remnants of stellar evolution, the collapsed cores of massive stars. They are compact objects that challenge many of our current theories of how matter can exist at densities similar to that of the atomic nucleus. Pulsars, highly magnetic neutron stars, can provide some information through emission of radiation. Particularly, many observed X-ray pulsars radiate due to the accumulation of material from a companion star in a binary system. This process of accretion in the presence of an extreme magnetic field results in the formation of plasma funnels at the pulsar’s magnetic poles. Inside these extremely hot accretion columns is where the X-ray continuum is produced. In addition, the X-ray continuum of an accreting pulsar may show a feature, known as a cyclotron line, which provides a direct measurement of the neutron star’s magnetic field. Historically and to this day, the studies of the accreting pulsar continuum have been performed by using standard phenomenological cutoff power-law shaped models that provide no physical description. However, in the past few years, physically descriptive models have been under development. For the work presented in this dissertation, I analyzed data from the Japanese X-ray satellite, Suzaku. I first performed a detailed temporal and standard spectral analysis of the accreting pulsar XTEJ1946+274. Building on that, I then conducted a self-consistent study of the X-ray emission of a sample of nine accreting pulsars. The first step involved examining the broadband X-ray continua with phenomenological models, where I successfully applied the Fermi-Dirac cutoff powerlaw model to all sources. I also investigated additional X-ray spectral features that in part originate outside the accretion column, such as binary-intrinsic absorption, fluorescence, blackbody emission, and others. The second step involved testing the application of a newly implemented physical model to the same pulsar sample, and thereby obtaining physical descriptions of the accretion column structure and geometry, i.e., radius, plasma (electron) temperature, and ratios of different Comptonization effects inside the accretion column. By comparing the physical and phenomenological spectral fit results, I provide first observational proof that the plasma temperature inside the accretion column is related to the degree of curvature of the X-ray continuum. Lastly, I present a remarkable self-consistency of the pulsar sample study by describing how the plasma temperature inside the accretion column, estimated from the thermal broadening effect of the cyclotron line, is consistent with the temperatures described by the physical model. To summarize, considerable progress has been made in recent years regarding the development of physical models describing the accretion process onto a highly magnetic neutron stars. I successfully tested one of these newly implemented models and provided the first direct connection between physical parameters of the accretion process (magnetic field strength, plasma temperature, plasma density, mass accretion rate) and the X-ray continuum spectral shape.
TITLE: Shedding New Light on Accreting Pulsars.
ABSTRACT: Neutron stars are remnants of stellar evolution, the collapsed cores of massive stars. They are compact objects that challenge many of our current theories of how matter can exist at densities similar to that of the atomic nucleus. Pulsars, highly magnetic neutron stars, can provide some information through emission of radiation. Particularly, many observed X-ray pulsars radiate due to the accumulation of material from a companion star in a binary system. This process of accretion in the presence of an extreme magnetic field results in the formation of plasma funnels at the pulsar’s magnetic poles. Inside these extremely hot accretion columns is where the X-ray continuum is produced. In addition, the X-ray continuum of an accreting pulsar may show a feature, known as a cyclotron line, which provides a direct measurement of the neutron star’s magnetic field. Historically and to this day, the studies of the accreting pulsar continuum have been performed by using standard phenomenological cutoff power-law shaped models that provide no physical description. However, in the past few years, physically descriptive models have been under development. For the work presented in this dissertation, I analyzed data from the Japanese X-ray satellite, Suzaku. I first performed a detailed temporal and standard spectral analysis of the accreting pulsar XTEJ1946+274. Building on that, I then conducted a self-consistent study of the X-ray emission of a sample of nine accreting pulsars. The first step involved examining the broadband X-ray continua with phenomenological models, where I successfully applied the Fermi-Dirac cutoff powerlaw model to all sources. I also investigated additional X-ray spectral features that in part originate outside the accretion column, such as binary-intrinsic absorption, fluorescence, blackbody emission, and others. The second step involved testing the application of a newly implemented physical model to the same pulsar sample, and thereby obtaining physical descriptions of the accretion column structure and geometry, i.e., radius, plasma (electron) temperature, and ratios of different Comptonization effects inside the accretion column. By comparing the physical and phenomenological spectral fit results, I provide first observational proof that the plasma temperature inside the accretion column is related to the degree of curvature of the X-ray continuum. Lastly, I present a remarkable self-consistency of the pulsar sample study by describing how the plasma temperature inside the accretion column, estimated from the thermal broadening effect of the cyclotron line, is consistent with the temperatures described by the physical model. To summarize, considerable progress has been made in recent years regarding the development of physical models describing the accretion process onto a highly magnetic neutron stars. I successfully tested one of these newly implemented models and provided the first direct connection between physical parameters of the accretion process (magnetic field strength, plasma temperature, plasma density, mass accretion rate) and the X-ray continuum spectral shape.
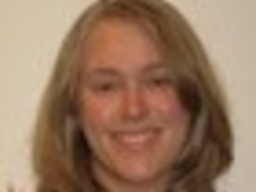